SIZE AND SHAPE OF THE EARTH
Moon and earth cores While the Earth appears to be round when viewed from the vantage point of space, it is actually closer to an ellipsoid. Technically The Earth is an oblate ellipsoid, an ellipse rotated about its minor axis, with an equatorial radius of 6,378.1 kilometers (3963 miles), and equatorial of diameter of 12,742 kilometers (7917 miles) and an equatorial circumference of 40,075 kilometers (24,901 miles), which is slightly greater than the polar radius of 6,356.7 kilometers (3,950 miles), the polar diameter of 12,713.6 kilometers (7900 miles) and polar circumference of 40,008 kilometers (24,860 miles). The small equatorial bulge is due to earth’s rotation. [Source: Robert Stewart, “Introduction to Physical Oceanography”, Texas A&M University, 2008]
However, even an ellipsoid does not adequately describe the Earth’s unique and ever-changing shape. The Earthis pudgier at the equator than at the poles by about 21.3 kilometers (13.2 miles) due to the centrifugal force created by the earth’s constant rotation. Mountains rising to over nin kilometers (5.7 miles) and ocean trenches diving to 11 kilometers (6.8 miles) below sea level further distort the shape of the Earth. Sea level itself is even irregularly shaped. Slight variations in Earth’s gravity field cause permanent hills and valleys in the ocean’s surface of over 91.5 meters (300 feet) relative to an ellipsoid. [Source: NOAA]
Additionally, the shape of the Earth is always changing. Sometimes this change is periodic, as is the case with daily tides that affect both the ocean and the crust; sometimes the change is slow and steady, as with the drift of tectonic plates or the rebound of the crust after a heavy sheet of ice has melted; and sometimes the shape of the planet changes in violent, episodic ways during events such as earthquakes, volcanic eruptions, or meteor strikes. The National Geodetic Survey measures and monitors our ever-changing planet. Geodesy is the science of measuring and monitoring the size and shape of the Earth, including its gravity field, and determining the location of points on the Earth’s surface.
Measurements of the Earth
Distances on earth are measured using different units, the most common are degrees of latitude or longitude, kilometers, meters, feet, miles, and nautical miles. Latitude is the angle between the local vertical and the equatorial plane. A meridian is the intersection at earth’s surface of a plane perpendicular to the equatorial plane and passing through earth’s axis of rotation. Longitude is the angle between the standard meridian and any other meridian, where the standard meridian is the one that passes through a point at the Royal Observatory at Greenwich, England. Thus longitude is measured east or west of Greenwich. A degree of latitude is not the same length as a degree of longitude except at the equator. [Source: Robert Stewart, “Introduction to Physical Oceanography”, Texas A&M University, 2008]
Latitude and longitude have been used in astronomy and navigation since ancient times. By calculating the angle between the horizon and a celestial object (usually the sun or the North Star), navigators could determine their approximate latitude using basic tools. The calculations were simple, so measuring latitude at sea was reliable hundreds of years before accurate longitude measurements could be calculated during a voyage. If the North Star was 60 degrees above the horizon, the observer was at 60 degrees latitude (north). This process was more complex in the southern hemisphere, where the North Star is not visible. [Source: NOAA]
We still look to the sky to determine our position, but the equipment is a bit more sophisticated. A constellation of over 30 global positioning satellites orbit the Earth, transmitting signals to receivers on land. NOS’s National Geodetic Survey manages a network of stationary global positioning satellite receivers called Continuously Operating Reference Stations (CORS). When combined with other positioning data in the National Spatial Reference System, processed CORS data can provide latitude, longitude, and height positions accurate to within a few centimeters.
International Date Line serves as the "line of demarcation" between two consecutive calendar dates. Established in 1884, it passes through the mid-Pacific Ocean and roughly follows a 180 degrees longitude north-south line on the Earth. It is located halfway around the world from the prime meridian — the 0 degrees longitude line in Greenwich, England. When you cross the date line, you become a time traveler of sorts! Cross to the west and it’s one day later; cross back and you’ve “gone back in time." Despite its name, the international date line has no legal international status and countries are free to choose the dates that they observe. While the date line generally runs north to south from pole to pole, it zigzags around political borders such as eastern Russia and Alaska’s Aleutian Islands. [Source: NOAA]
Latitude
Earth Layers Lines of latitude, also called parallels, are imaginary lines that divide the Earth. They run east to west, but measure your distance north or south. The equator is the most well known parallel. At 0 degrees latitude, it equally divides the Earth into the Northern and Southern hemispheres. From the equator, latitude increases as you travel north or south, reaching 90 degrees at each pole. [Source: NOAA]
There are other named lines of latitude. They’re based on the sun’s position during Earth’s orbit, and they help us understand climate, weather, and ocean currents. The Tropic of Cancer, at roughly 23 degrees north, and the Tropic of Capricorn, at roughly 23 degrees south, are the boundaries of what we consider the tropics. The Arctic Circle and the Antarctic Circle are at roughly 66 degrees north and south, respectively. They mark the boundaries of the Arctic and Antarctic regions.
Each degree of latitude covers about 111 kilometers on the Earth’s surface. One degree of latitude can be further divided into 60 minutes, and one minute can be further divided into 60 seconds. A second of latitude covers only about 30.7 meters. Unlike longitude lines, which get closer to each other at the poles, latitude lines are parallel. No matter where you are on Earth, latitude lines are the same distance apart.
If we assume the Earth is a perfect sphere, lines of latitude are relatively simple to calculate. The latitude of a certain point on the surface of the Earth is the angle between two lines: a line from that location to the center of the Earth and a line from the center of the Earth to the Equator. This method of calculation gives us geocentric lines of latitude. However, this method is not accurate enough for astronomy, global positioning, and other real-world applications. Instead, we use what is known as geodetic latitude. This method of calculating latitude for any point on Earth accounts for the fact that the Earth is actually squished at the poles due to the centrifugal force created by the planet's rotation. Measuring latitude lines with high degrees of accuracy is but one component of geodesy, the science of accurately measuring and understanding the Earth's geometric shape, orientation in space, and gravity field.
Longitude
Lines of longitude, also called meridians, are imaginary lines that divide the Earth. They run north to south from pole to pole, but they measure the distance east or west. The prime meridian, which runs through Greenwich, England, has a longitude of 0 degrees. It divides the Earth into the eastern and western hemispheres. The antimeridian is on the opposite side of the Earth, at 180 degrees longitude. Though the antimeridian is the basis for the international date line, actual date and time zone boundaries are dependent on local laws. The international date line zigzags around borders near the antimeridian. [Source: NOAA]
Like latitude, longitude is measured in degrees, minutes, and seconds. Although latitude lines are always equally spaced, longitude lines are furthest from each other at the equator and meet at the poles. At the equator, longitude lines are the same distance apart as latitude lines — one degree covers about 111 kilometers (69 miles). But, by 60 degrees north or south, that distance is down to 56 kilometers (35 miles). By 90 degrees north or south (at the poles), it reaches zero.
Unlike the equator (which is halfway between the Earth’s north and south poles), the prime meridian is an arbitrary line. In 1884, representatives at the International Meridian Conference in Washington, D.C., met to define the meridian that would represent 0 degrees longitude. For its location, the conference chose a line that ran through the telescope at the Royal Observatory in Greenwich, England. At the time, many nautical charts and time zones already used Greenwich as the starting point, so keeping this location made sense. But, if you go to Greenwich with your GPS receiver, you’ll need to walk 102 meters (334 feet) east of the prime meridian markers before your GPS shows 0 degrees longitude. In the 19th century, scientists did not take into account local variations in gravity or the slightly squished shape of the Earth when they determined the location of the prime meridian. Satellite technology, however, allows scientists to more precisely plot meridians so that they are straight lines running north and south, unaffected by local gravity changes. In the 1980s, the International Reference Meridian (IRM) was established as the precise location of 0 degrees longitude. Unlike the prime meridian, the IRM is not a fixed location, but will continue to move as the Earth’s surface shifts.
Determining Longitude
Igneous structures Navigators and mariners have been able to measure latitude with basic tools for thousands of years. Longitude, however, required more advanced tools and calculations. Starting in the 16th century, European governments began offering huge rewards if anyone could solve “the longitude problem.” Several methods were tried, but the best and simplest way to measure longitude from a ship was with an accurate clock. [Source: NOAA]
A navigator would compare the time at local noon (when the sun is at its highest point in the sky) to an onboard clock that was set to Greenwich Mean Time (the time at the prime meridian). Each hour of difference between local noon and the time in Greenwich equals 15 degrees of longitude. Why? Because the Earth rotates 360 degrees in 24 hours, or 15 degrees per hour. If the sun’s position tells the navigator it’s local noon, and the clock says back in Greenwich, England, it’s 2 p.m., the two-hour difference means the ship’s longitude is 30 degrees west.
But aboard a swaying ship in varying temperatures and salty air, even the most accurate clocks of the age did a poor job of keeping time. It wasn’t until marine chronometers were invented in the 18th century that longitude could be accurately measured at sea.
Accurate clocks are still critical to determining longitude, but now they’re found in GPS satellites and stations. Each GPS satellite is equipped with one or more atomic clocks that provide incredibly precise time measurements, accurate to within 40 nanoseconds (or 40 billionths of a second). The satellites broadcast radio signals with precise timestamps. The radio signals travel at a constant speed (the speed of light), so we can easily calculate the distance between a satellite and GPS receiver if we know precisely how long it took for the signal to travel between them.
On the ground, NOAA’s National Geodetic Survey manages the Continuously Operating Reference Stations Network, which comprises 1,800 stationary, permanently operating GPS stations. These CORS continuously receive GPS radio signals and incorporate that data into the National Spatial Reference System. The GPS position on a smartphone is accurate to within about 5 meters (16 feet), but processed CORS data can provide longitude accurate to within a few centimeters, along with latitude and height positions.
GPS
The Global Positioning System (GPS) lets us know where we are (and where we are going) anywhere on Earth as determined by various generations of GPS satellites orbiting the Earth such as the GPS Block IIR(M) Satellite. GPS satellites fly in medium Earth orbit at an altitude of approximately 20,200 Kilometers. Each satellite circles the Earth twice a day. The satellites in the GPS constellation are arranged so that users can view at least four satellites from virtually any point on the planet. [Source: NOAA]
GPS is a three-part system: satellites, ground stations, and receivers. Satellites act like the stars in constellations — we know where they are supposed to be at any given time. The ground stations monitor and control the satellites, and they help determine their locations — both where they were and where they are forecast to be. A receiver, like you might find in your phone or in your car, is constantly listening for signals from these satellites, which can be used like a giant tape measure between the receiver and satellites.
Once your receiver calculates its distance from four or more satellites, it knows exactly where you are. Within seconds, from thousands of miles up in space, your location on the ground can be determined with incredible precision, often within a few yards of your actual location. Many high-tech receivers can figure out where you are to within a fraction of an inch.
Lidar
Lidar, which stands for Light Detection and Ranging, is a remote sensing method that uses light in the form of a pulsed laser to measure ranges (variable distances) to the Earth. These light pulses — combined with other data recorded by the airborne system — generate precise, three-dimensional information about the shape of the Earth and its surface characteristics. [Source: NOAA]
A lidar instrument principally consists of a laser, a scanner, and a specialized GPS receiver. Airplanes and helicopters are the most commonly used platforms for acquiring lidar data over broad areas. Two types of lidar are topographic and bathymetric. Topographic lidar typically uses a near-infrared laser to map the land, while bathymetric lidar uses water-penetrating green light to also measure seafloor and riverbed elevations.
Lidar systems allow scientists and mapping professionals to examine both natural and manmade environments with accuracy, precision, and flexibility. NOAA scientists are using lidar to produce more accurate shoreline maps, make digital elevation models for use in geographic information systems, to assist in emergency response operations, and in many other applications.
Geodesy
Geodesy is the science of accurately measuring and understanding three fundamental properties of the Earth: its geometric shape, its orientation in space, and its gravity field — as well as the changes of these properties with time. By using GPS, geodesists can monitor the movement of a site 24 hours a day, seven days a week. [Source: NOAA]
Many organizations use geodesy to map the U.S. shoreline, determine land boundaries, and improve transportation and navigation safety. To measure points on the Earth’s surface, geodesists assign coordinates (similar to a unique address) to points all over the Earth. In the past, geodesists determined the coordinates of points by using Earth-based surveying tools to measure the distances between points. Today, geodesists use space-based tools like the Global Positioning System (GPS) to measure points on the Earth’s surface.
Geodesists must accurately define the coordinates of points on the surface of the Earth in a consistent manner. A set of accurately measured points is the basis for the National Spatial Reference System, which allows different kinds of maps to be consistent with one another.
To measure the Earth, geodesists build simple mathematical models of the Earth which capture the largest, most obvious features. Geodesists have adopted the ellipsoid as the most basic model of the Earth. Because the ellipsoid is based on a very simple mathematical model, it can be completely smooth and does not include any mountains or valleys. When additional detail of the Earth is needed, geodesists use the geoid. A geoid has a shape very similar to global mean sea level, but this exists over the whole globe, not just over the oceans.
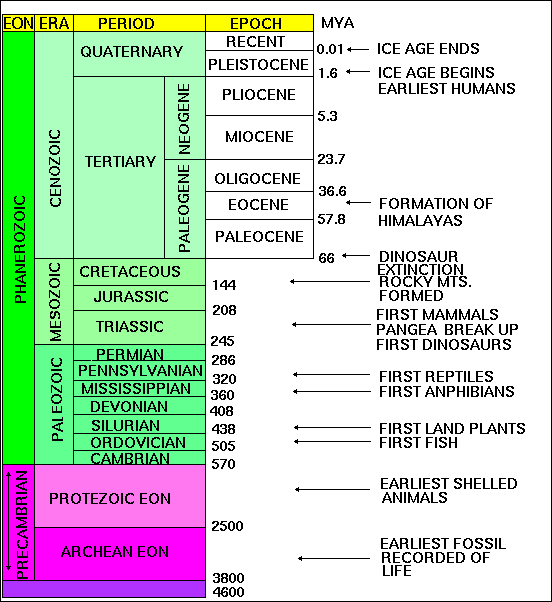
Geologic Time Scale
Age of the Earth
The Earth is very old — 4.5 billion years or more — according to recent estimates. This vast span of time, called geologic time by earth scientists, is difficult to comprehend in the familiar time units of months and years, or even centuries. How then do scientists reckon geologic time, and why do they believe the Earth is so old? A great part of the secret of the Earth's age is locked up in its rocks, and our centuries-old search for the key led to the beginning and nourished the growth of geologic science.
Ancient rocks exceeding 3.5 billion years in age are found on all of Earth's continents. The oldest rocks on Earth found so far are the Acasta Gneisses in northwestern Canada near Great Slave Lake (4.03 Ga) and the Isua Supracrustal rocks in West Greenland (3.7 to 3.8 Ga), but well-studied rocks nearly as old are also found in the Minnesota River Valley and northern Michigan (3.5-3.7 billion years), in Swaziland (3.4-3.5 billion years), and in Western Australia (3.4-3.6 billion years). These ancient rocks have been dated by a number of radiometric dating methods and the consistency of the results give scientists confidence that the ages are correct to within a few percent.
An interesting feature of these ancient rocks is that they are not from any sort of "primordial crust" but are lava flows and sediments deposited in shallow water, an indication that Earth history began well before these rocks were deposited. In Western Australia, single zircon crystals found in younger sedimentary rocks have radiometric ages of as much as 4.3 billion years, making these tiny crystals the oldest materials to be found on Earth so far. The source rocks for these zircon crystals have not yet been found. The ages measured for Earth's oldest rocks and oldest crystals show that the Earth is at least 4.3 billion years in age but do not reveal the exact age of Earth's formation. The best age for the Earth (4.54 Ga) is based on old, presumed single-stage leads coupled with the Pb ratios in troilite from iron meteorites, specifically the Canyon Diablo meteorite. In addition, mineral grains (zircon) with U-Pb ages of 4.4 Ga have recently been reported from sedimentary rocks in west-central Australia.
Determining the Age of the Earth
So far scientists have not found a way to determine the exact age of the Earth directly from Earth rocks because Earth's oldest rocks have been recycled and destroyed by the process of plate tectonics. If there are any of Earth's primordial rocks left in their original state, they have not yet been found. Nevertheless, scientists have been able to determine the probable age of the Solar System and to calculate an age for the Earth by assuming that the Earth and the rest of the solid bodies in the Solar System formed at the same time and are, therefore, of the same age.
The ages of Earth and Moon rocks and of meteorites are measured by the decay of long-lived radioactive isotopes of elements that occur naturally in rocks and minerals and that decay with half lives of 700 million to more than 100 billion years to stable isotopes of other elements. These dating techniques, which are firmly grounded in physics and are known collectively as radiometric dating, are used to measure the last time that the rock being dated was either melted or disturbed sufficiently to rehomogenize its radioactive elements.
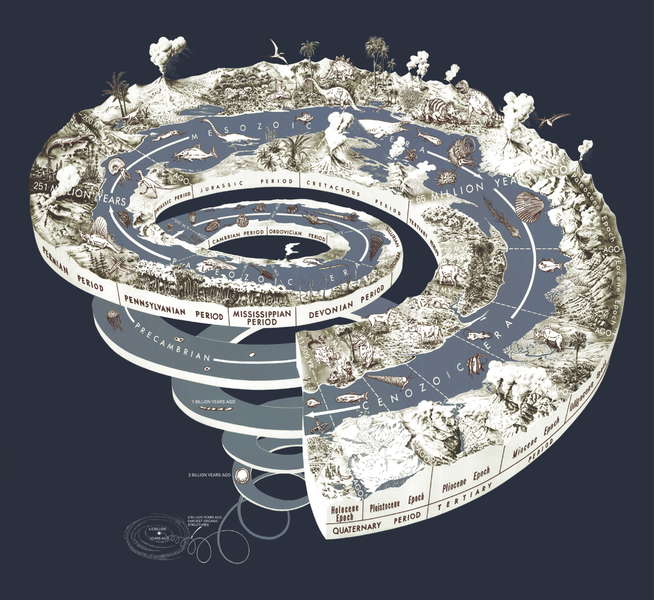
Geological time spiral
The Moon is a more primitive planet than Earth because it has not been disturbed by plate tectonics; thus, some of its more ancient rocks are more plentiful. Only a small number of rocks were returned to Earth by the six Apollo and three Luna missions. These rocks vary greatly in age, a reflection of their different ages of formation and their subsequent histories. The oldest dated moon rocks, however, have ages between 4.4 and 4.5 billion years and provide a minimum age for the formation of our nearest planetary neighbor. Thousands of meteorites, which are fragments of asteroids that fall to Earth, have been recovered. These primitive objects provide the best ages for the time of formation of the Solar System. There are more than 70 meteorites, of different types, whose ages have been measured using radiometric dating techniques. The results show that the meteorites, and therefore the Solar System, formed between 4.53 and 4.58 billion years ago. The best age for the Earth comes not from dating individual rocks but by considering the Earth and meteorites as part of the same evolving system in which the isotopic composition of lead, specifically the ratio of lead-207 to lead-206 changes over time owing to the decay of radioactive uranium-235 and uranium-238, respectively.
Scientists have used this approach to determine the time required for the isotopes in the Earth's oldest lead ores, of which there are only a few, to evolve from its primordial composition, as measured in uranium-free phases of iron meteorites, to its compositions at the time these lead ores separated from their mantle reservoirs. These calculations result in an age for the Earth and meteorites, and hence the Solar System, of 4.54 billion years with an uncertainty of less than 1 percent. To be precise, this age represents the last time that lead isotopes were homogeneous througout the inner Solar System and the time that lead and uranium was incorporated into the solid bodies of the Solar System. The age of 4.54 billion years found for the Solar System and Earth is consistent with current calculations of 11 to 13 billion years for the age of the Milky Way Galaxy (based on the stage of evolution of globular cluster stars) and the age of 10 to 15 billion years for the age of the Universe (based on the recession of distant galaxies).
Evolution of Geology and Ideas About Geologic Time
Mankind's speculations about the nature of the Earth inspired much of the lore and legend of early civilizations, but at times there were flashes of insight. The ancient historian Herodotus, in the 5th century B.C., made one of the earliest recorded geological observations. After finding fossil shells far inland in what are now parts of Egypt and Libya, he correctly inferred that the Mediterranean Sea had once extended much farther to the south. Few believed him, however, nor did the idea catch on. In the 3rd century B.C., Eratosthenes depicted a spherical Earth and even calculated its diameter and circumference, but the concept of a spherical Earth was beyond the imagination of most men. Only 500 years ago, sailors aboard the Santa Maria begged Columbus to turn back lest they sail off the Earth's "edge." Similar opinions and prejudices about the nature and age of the Earth have waxed and waned through the centuries. Most people, however, appear to have traditionally believed the Earth to be quite young — that its age might be measured in terms of thousands of years, but certainly not in millions.
The evidence for an ancient Earth is concealed in the rocks that form the Earth's crust and surface. The rocks are not all the same age — or even nearly so — but, like the pages in a long and complicated history, they record the Earthshaping events and life of the past. The record, however, is incomplete. Many pages, especially in the early parts, are missing and many others are tattered, torn, and difficult to decipher. But enough of the pages are preserved to reward the reader with accounts of astounding episodes which certify that the Earth is billions of years old.
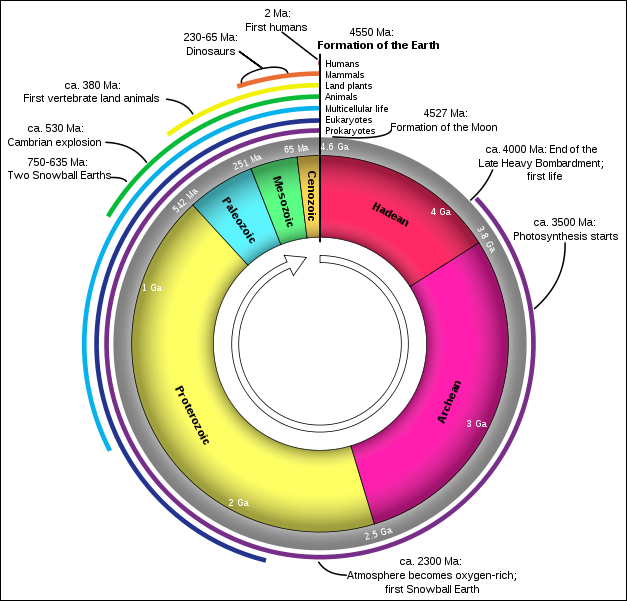
Geologic clock with events and periods
Two scales are used to date these episodes and to measure the age of the Earth: a relative time scale, based on the sequence of layering of the rocks and the evolution of life, and the radiometric time scale, based on the natural radioactivity of chemical elements in some of the rocks. An explanation of the relative scale highlights events in the growth of geologic science itself; the radiometric scale is a more recent development borrowed from the physical sciences and applied to geologic problems.
At the close of the 18th century, the haze of fantasy and mysticism that tended to obscure the true nature of the Earth was being swept away. Careful studies by scientists showed that rocks had diverse origins. Some rock layers, containing clearly identifiable fossil remains of fish and other forms of aquatic animal and plant life, originally formed in the ocean. Other layers, consisting of sand grains winnowed clean by the pounding surf, obviously formed as beach deposits that marked the shorelines of ancient seas. Certain layers are in the form of sand bars and gravel banks — rock debris spread over the land by streams. Some rocks were once lava flows or beds of cinders and ash thrown out of ancient volcanoes; others are portions of large masses of once molten rock that cooled very slowly far beneath the Earth's surface. Other rocks were so transformed by heat and pressure during the heaving and buckling of the Earth's crust in periods of mountain building that their original features were obliterated.
Between the years of 1785 and 1800, James Hutton and William Smith advanced the concept of geologic time and strengthened the belief in an ancient world. Hutton, a Scottish geologist, first proposed formally the fundamental principle used to classify rocks according to their relative ages. He concluded, after studying rocks at many outcrops, that each layer represented a specific interval of geologic time. Further, he proposed that wherever uncontorted layers were exposed, the bottom layer was deposited first and was, therefore, the oldest layer exposed; each succeeding layer, up to the topmost one, was progressively younger.
Today, such a proposal appears to be quite elementary but, nearly 200 years ago, it amounted to a major breakthrough in scientific reasoning by establishing a rational basis for relative time measurements. However, unlike tree-ring dating — in which each ring is a measure of 1 year's growth — no precise rate of deposition can be determined for most of the rock layers. Therefore, the actual length of geologic time represented by any given layer is usually unknown or, at best, a matter of opinion.
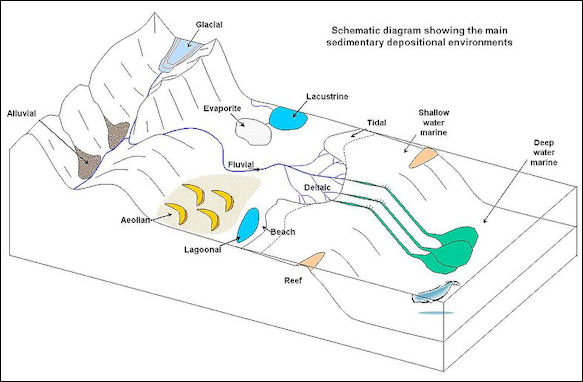
Sedimentary environment
Rock Strata and Geologic Time
William "Strata" Smith, a civil engineer and surveyor, was well acquainted with areas in southern England where "limestone and shales are layered like slices of bread and butter." His hobby of collecting and cataloging fossil shells from these rocks led to the discovery that certain layers contained fossils unlike those in other layers. Using these key or index fossils as markers, Smith could identify a particular layer of rock wherever it was exposed. Because fossils actually record the slow but progressive development of life, scientists use them to identify rocks of the same age throughout the world.
From the results of studies on the origins of the various kinds of rocks (petrology), coupled with studies of rock layering (stratigraphy) and the evolution of life (paleontology), geologists reconstruct the sequence of events that has shaped the Earth's surface. Their studies show, for example, that during a particular episode the land surface was raised in one part of the world to form high plateaus and mountain ranges. After the uplift of the land, the forces of erosion attacked the highlands and the eroded rock debris was transported and redeposited in the lowlands. During the same interval of time in another part of the world, the land surface subsided and was covered by the seas. With the sinking of the land surface, sediments were deposited on the ocean floor. The evidence for the pre-existence of ancient mountain ranges lies in the nature of the eroded rock debris, and the evidence of the seas' former presence is, in part, the fossil forms of marine life that accumulated with the bottom sediments.
Such recurring events as mountain building and sea encroachment, of which the rocks themselves are records, comprise units of geologic time even though the actual dates of the events are unknown. By comparison, the history of mankind is similarly organized into relative units of time. We speak of human events as occurring either B.C. or A.D. — broad divisions of time. Shorter spans are measured by the dynasties of ancient Egypt or by the reigns of kings and queens in Europe. Geologists have done the same thing to geologic time by dividing the Earth's history into Eras — broad spans based on the general character of life that existed during these times — and Periods — shorter spans based partly on evidence of major disturbances of the Earth's crust.
The names used to designate the divisions of geologic time are a fascinating mixture of words that mark highlights in the historical development of geologic science over the past 200 years. Nearly every name signifies the acceptance of a new scientific concept — a new rung in the ladder of geologic knowledge.
The Grand Canyon is only five million years old. Many think of it as being older than that as the rock that is exposed is tens or even hundreds of millions of years old. The Grand Canyon gets its stunning colors from sand that originated from the billion-year-old Appalachian mountains 2,000 miles away. As sand eroded from the Appalachians it was carried westward by massive rivers.
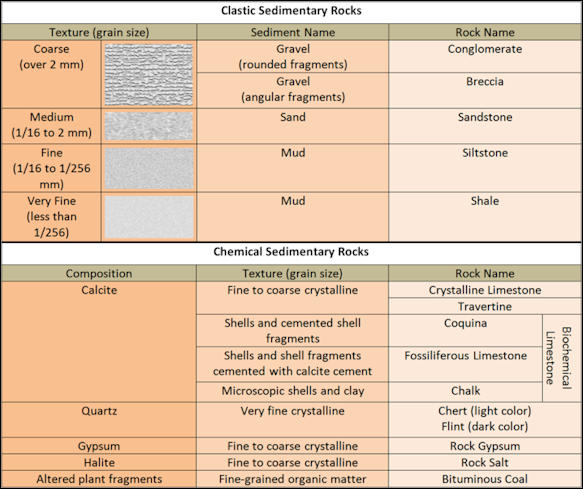
Sedimentary Rock Chart
Dating Rocks by Radioactive Decay
The discovery of the natural radioactive decay of uranium in 1896 by Henry Becquerel, the French physicist, opened new vistas in science. In 1905, the British physicist Lord Rutherford — after defining the structure of the atom — made the first clear suggestion for using radioactivity as a tool for measuring geologic time directly; shortly thereafter, in 1907, Professor B. B. Boltwood, radiochemist of Yale Uniyersity, published a list of geologic ages based on radioactivity. Although Boltwood's ages have since been revised, they did show correctly that the duration of geologic time would be measured in terms of hundreds-to-thousands of millions of years.
The next 40 years was a period of expanding research on the nature and behavior of atoms, leading to the development of nuclear fission and fusion as energy sources. A byproduct of this atomic research has been the development and continuing refinement of the various methods and techniques used to measure the age of Earth materials. Precise dating has been accomplished since 1950.
A chemical element consists of atoms with a specific number of protons in their nuclei but different atomic weights owing to variations in the number of neutrons. Atoms of the same element with differing atomic weights are called isotopes. Radioactive decay is a spontaneous process in which an isotope (the parent) loses particles from its nucleus to form an isotope of a new element (the daughter). The rate of decay is conveniently expressed in terms of an isotope's half-life, or the time it takes for one-half of a particular radioactive isotope in a sample to decay. Most radioactive isotopes have rapid rates of decay (that is, short half-lives) and lose their radioactivity within a few days or years. Some isotopes, however, decay slowly, and several of these are used as geologic clocks. The parent isotopes and corresponding daughter products most commonly used to determine the ages of ancient rocks are listed below:
Major Parent Isotopes used to date rocks (Stable Daughter Product, Currently Accepted Half-Life Values): 1) Uranium-238 (Lead-206, 4.5 billion years); 2) Uranium-235 (Lead-207, 704 million years); 3) Thorium-232 (Lead-208, 14.0 billion years); 4) Rubidium-87 (Strontium-87, 48.8 billion years); 5) Potassium-40 (Argon-40, 1.25 billion years); 6) (Samarium-147, Neodymium-143, 106 billion years).
Dating rocks by these radioactive timekeepers is simple in theory, but the laboratory procedures are complex. The numbers of parent and daughter isotopes in each specimen are determined by various kinds of analytical methods. The principal difficulty lies in measuring precisely very small amounts of isotopes.
The potassium-argon method can be used on rocks as young as a few thousand years as well as on the oldest rocks known. Potassium is found in most rock-forming minerals, the half-life of its radioactive isotope potassium-40 is such that measurable quantities of argon (daughter) have accumulated in potassium-bearing minerals of nearly all ages, and the amounts of potassium and argon isotopes can be measured accurately, even in very small quantities. Where feasible, two or more methods of analysis are used on the same specimen of rock to confirm the results.
Another important atomic clock used for dating purposes is based on the radioactive decay of the isotope carbon-14, which has a half-life of 5,730 years. Carbon-14 is produced continuously in the Earth's upper atmosphere as a result of the bombardment of nitrogen by neutrons from cosmic rays. This newly formed radiocarbon becomes uniformly mixed with the nonradioactive carbon in the carbon dioxide of the air, and it eventually finds its way into all living plants and animals. In effect, all carbon in living organisms contains a constant proportion of radiocarbon to nonradioactive carbon. After the death of the organism, the amount of radiocarbon gradually decreases as it reverts to nitrogen-14 by radioactive decay. By measuring the amount of radioactivity remaining in organic materials, the amount of carbon-14 in the materials can be calculated and the time of death can be determined. For example, if carbon from a sample of wood is found to contain only half as much carbon-14 as that from a living plant, the estimated age of the old wood would be 5,730 years.
The radiocarbon clock has become an extremely useful and efficient tool in dating the important episodes in the recent prehistory and history of man, but because of the relatively short half-life of carbon-14, the clock can be used for dating events that have taken place only within the past 50,000 years.
Interweaving the relative time scale with the atomic time scale poses certain problems because only certain types of rocks, chiefly the igneous variety, can be dated directly by radiometric methods; but these rocks do not ordinarily contain fossils. Igneous rocks are those such as granite and basalt which crystallize from molten material called "magma".
When igneous rocks crystallize, the newly formed minerals contain various amounts of chemical elements, some of which have radioactive isotopes. These isotopes decay within the rocks according to their half-life rates, and by selecting the appropriate minerals (those that contain potassium, for instance) and measuring the relative amounts of parent and daughter isotopes in them, the date at which the rock crystallized can be determined. Most of the large igneous rock masses of the world have been dated in this manner.
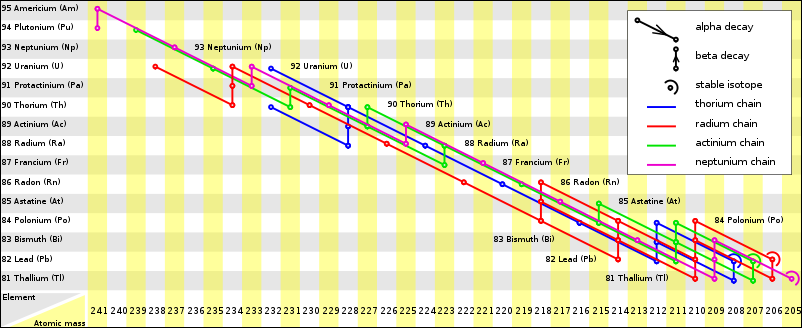
Radioactive decay chains diagram
Most sedimentary rocks such as sandstone, limestone, and shale are related to the radiometric time scale by bracketing them within time zones that are determined by dating appropriately selected igneous rocks, as shown by a hypothetical example.
Literally thousands of dated materials are now available for use to bracket the various episodes in the history of the Earth within specific time zones. Many points on the time scale are being revised, however, as the behavior of isotopes in the Earth's crust is more clearly understood. Thus the graphic illustration of the geologic time scale, showing both relative time and radiometric time, represents only the present state of knowledge. Certainly, revisions and modifications will be forthcoming as research continues to improve our knowledge of Earth history.
Image Sources: Wikimedia Commons, United States Geological Survey (USGS)
Text Sources: Mostly from USGS, CliffNotes (Weathering and Soil) and articles from the New York Times, Washington Post, Los Angeles Times, Times of London, Yomiuri Shimbun, The Guardian, National Geographic, The New Yorker, Time, Newsweek, Reuters, AP, Lonely Planet Guides, Compton’s Encyclopedia and various books and other publications.
Last updated February 2023